Clay minerals of natural soils are made up of silicon, aluminium, and/or iron and magnesium. They often have flat, layered structures with different shapes. Each clay particle in an expansive soil acts like a thin plate with negative charges on its surface and positive charges on its sides. The composition of clay can be thought of as different combinations of two basic building blocks. These building blocks are presented as simplified models for understanding the minerals’ structures.
Expansive soils, characterized by their significant volume changes (heave) upon wetting and shrinkage upon drying, constitute a major source of concern for civil engineering projects, impacting infrastructure stability and durability. These soils present complex geotechnical challenges due to their diverse mineralogical composition, sensitivity to moisture variations, and unpredictable behaviour under changing environmental conditions.
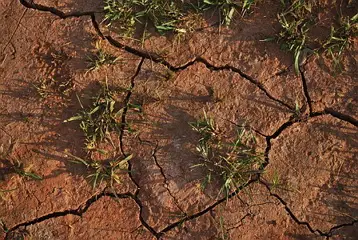
The primary clay mineral behind the expansive behaviour of these soils is the presence of smectite clays, particularly montmorillonite. These clays possess a 2:1 layered structure with interlayer spaces that readily accommodate water molecules, causing the layers to expand and the soil volume to increase. Upon drying, the interlayer water evaporates, resulting in layer contraction and soil shrinkage. This cyclic wetting and drying process can lead to significant differential movements, differential settlements, and the formation of desiccation cracks in expansive soils.
Therefore, clay minerals are an important microscopic factor affecting the engineering behaviour of soils. This article explores the common clay minerals and how their properties affect the expansive behaviour of soils.
Building Blocks of Clay Minerals
The building blocks have two basic elemental units: the silicon tetrahedron and the alumino-magnesium octahedron. They are shown in a simplified way in Figure 2. The silicon tetrahedron consists of silicon and oxygen atoms. Since silicon has a 4+ valence, it can bond with negative ions like oxygen (O2-) or hydroxyl (OH–), as seen in Figure 2(a). The relative sizes of the silicon and oxygen atoms make this structural unit take the shape of a tetrahedron.
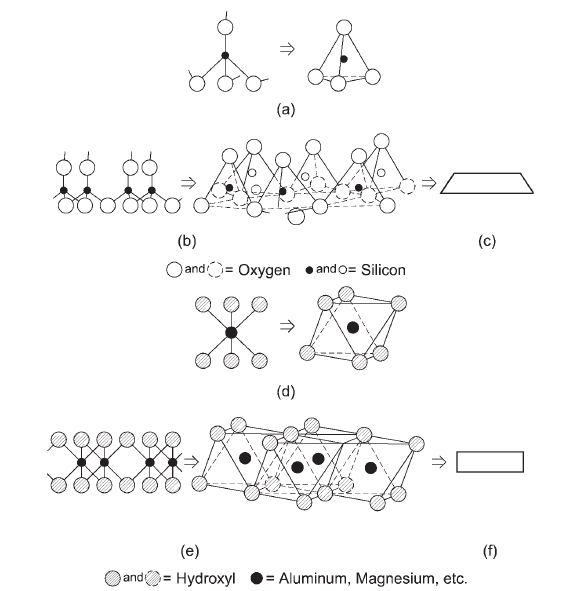
The alumino-magnesium octahedron is made up of aluminium or magnesium atoms with hydroxyls around them, as seen in Figure 2(d). These atoms are placed so that they can be imagined as forming an octahedral shape.
In the silicon tetrahedron in Figure 2(a), the oxygen atoms have an unfulfilled chemical bond each. The oxygen atoms at the bottom of a tetrahedron are shared with nearby tetrahedra, and the resulting arrangement of tetrahedra makes sheets, as seen in Figure 2(b). Sharing of oxygen atoms among the tetrahedra satisfies the oxygen atoms at the bottoms of the tetrahedra, but the oxygen atoms at the tops still have unsatisfied bonds, as seen in Figure 2(b). So, the top face of the silica sheet can form chemical bonds with positive cations.
The octahedral units share hydroxyls to make a sheet structure, as seen in Figure 2(e). The placement of the octahedral units is such that the hydroxyls in the sheet structure have fulfilled chemical bonds. The central cation in the octahedral sheet can change.
The building blocks that are used to show the crystalline structure of the different clay minerals are represented by schematic symbols in Figures 2(c) and 2(f). By changing the way these two building blocks are arranged, different clay minerals can be formed.
Types of Clay Minerals
The different minerals are grouped according to the order of the sheets. For this article, we will only consider three basic clay minerals:
- Kaolinite
- Illite
- Montmorillonite
Figure 3 shows simplified diagrams of the structures of these three minerals. The bonding between the different building blocks is very important for the behaviour of the different minerals.
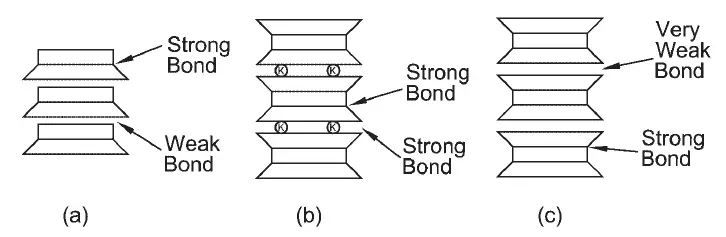
The term bentonite is often used for expansive soils. This term means clays that have a lot of montmorillonite. Bentonite is a very plastic, swelling clay material that mainly has montmorillonite. It is mined for business and is used for many things like drilling fluids, slurry trenches, cosmetics, paint thickeners, and more. Not all expansive soils are bentonite, but they are often called bentonite, usually by people who are not engineers.
Kaolinite Clay Mineral
Kaolinite group minerals feature a 1:1 layered structure. Each layer consists of a single tetrahedral silica sheet directly bonded to an octahedral alumina sheet. Unlike the 2:1 structure of smectites like montmorillonite, the absence of an intervening octahedral sheet results in stronger interlayer bonding and minimal swelling behaviour.
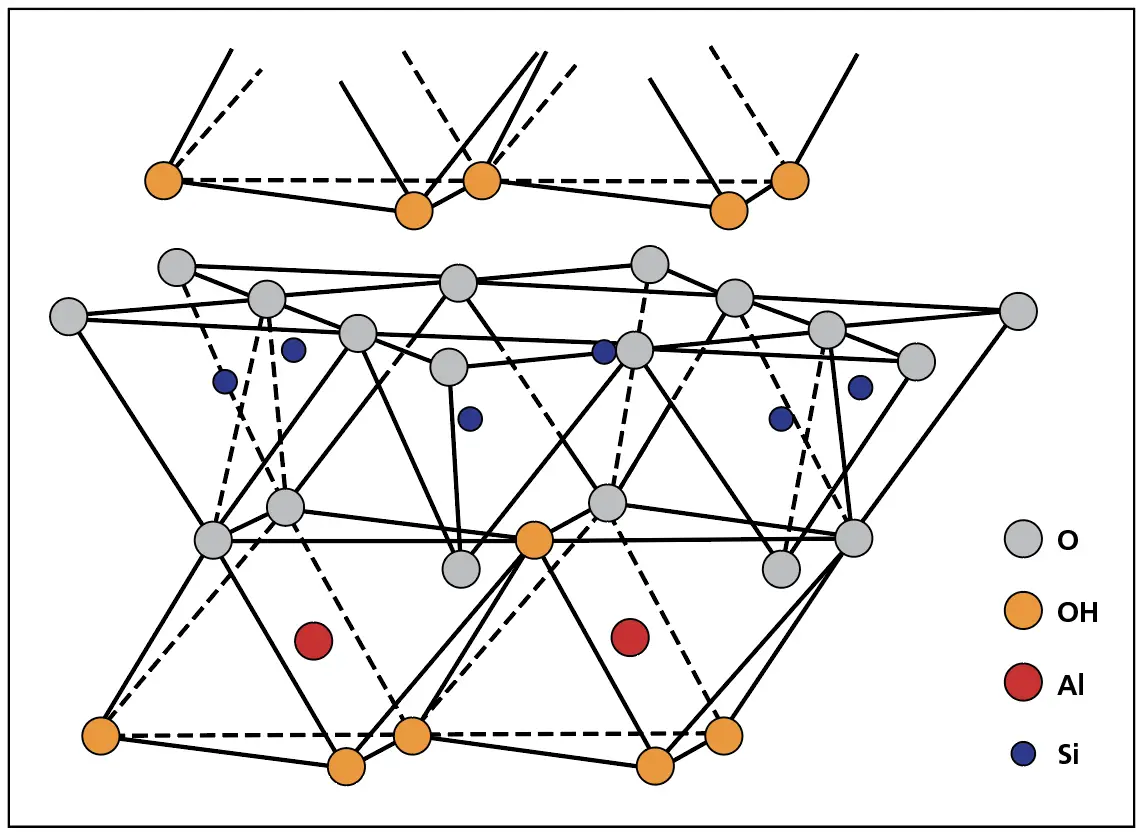
The bonds between the silica and octahedral sheets affect the size of the mineral particles in a soil. The kaolinite structure in Figure 4 shows that there is a strong bond between the octahedral sheet and the top of the silica sheet. This is because of how the atoms are arranged and the fact that some of the hydroxyls in the octahedral sheets can be replaced by the oxygen atoms at the top of the silica tetrahedra. So, there is a strong chemical bond between the octahedral sheet and the silica sheet.
In Figure 3a, the chemical bonds at the bottom of the silica sheet are satisfied, and so, the bond between the octahedral sheet and the bottom of the silica sheet is mainly formed by weaker hydrogen bonds. That is called a weak bond. So, when the mineral breaks, the sheets will split at the weak bond. However, the bonds are strong enough that the clay particles can have a number of building blocks in each clay particle. Therefore, the kaolinite particles are relatively big with a lateral size of up to 1 micron (μm) or more, and a thickness of 1/3 to 1/10 of the lateral size.
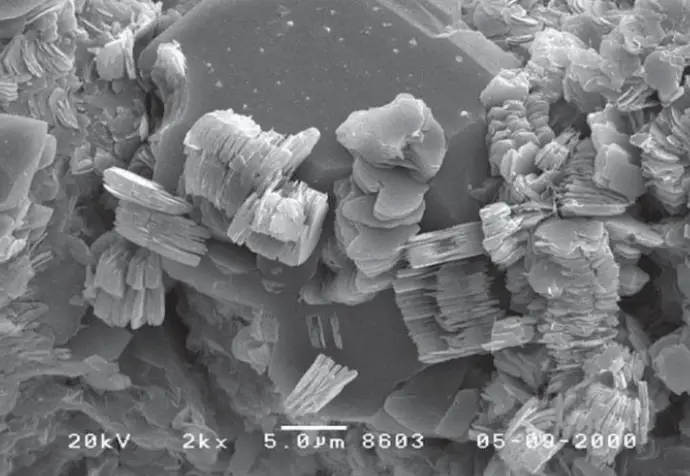
Characteristics of Kaolin Clay Mineral
- High chemical stability: Kaolinite exhibits exceptional resistance to weathering and chemical attack due to its strong covalent bonds and low reactivity.
- Low cation exchange capacity (CEC): Compared to smectites, kaolinite possesses a significantly lower CEC due to the absence of exchangeable cations in the interlayer space.
- High whiteness and brightness: The pure aluminium silicate composition and minimal iron impurities endow kaolinite with excellent whiteness and brightness, making it desirable for applications requiring high optical qualities.
- Good rheological properties: The platy morphology and surface properties of kaolinite contribute to its ability to thicken and suspend particles in aqueous suspensions, essential for its use in various industrial processes
Montmorillonite Clay Mineral
Montmorillonite is a member of the smectite family, a group of 2:1 layered silicates characterized by a tetrahedral silica sheet sandwiched between two octahedral alumina sheets. This layered structure gives rise to several unique properties, including a large surface area, high cation exchange capacity (CEC), and the ability to intercalate guest molecules between the silicate layers.
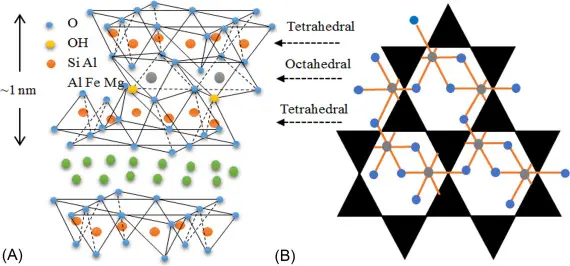
The bond between the two silica sheets at the bottom is made by weaker van der Waals forces for the montmorillonite, and it is marked as “very weak” in Figure 2c, unlike just “weak.” Because of this, montmorillonite particles may have only one or two sets of building blocks in thickness. So, the clay particles may be very thin, about 10 Ångström or less.
The unit cell of montmorillonite consists of two silica tetrahedral sheets linked to a central octahedral alumina sheet via shared oxygen atoms. This basic structure forms repeating layers stacked upon each other with weak van der Waals forces holding them together. The negative charge on the basal oxygen atoms is balanced by exchangeable cations (commonly Na+, Ca2+, or Mg2+) residing in the interlayer space. These cations contribute to the high CEC of montmorillonite, enabling its selective adsorption of various cations and organic molecules.
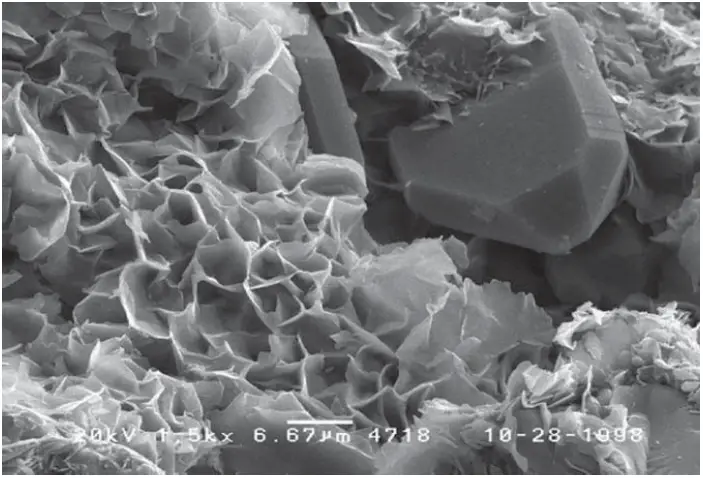
Characteristics of Montmorillonite
- Swelling: The presence of exchangeable cations and hydration of interlayer space allows montmorillonite to expand significantly upon contact with water, leading to increased volume and plasticity.
- Adsorption: The high surface area and CEC of montmorillonite facilitate the adsorption of various pollutants, toxins, and organic molecules, making it useful for environmental remediation and water purification.
- Cation exchange: The selectivity of montmorillonite towards specific cations can be exploited for soil amendment, nutrient retention, and catalysis.
- Intercalation: Guest molecules, such as polymers and drug molecules, can be inserted into the interlayer space of montmorillonite, leading to the formation of nanocomposites with unique properties.
Illite Clay Mineral
Illite comprises a group of mixed-layer clay minerals with structural features mirroring both smectites and micas. Its basic 2:1 layer unit resembles that of smectites, featuring a single tetrahedral silica sheet sandwiched between two octahedral alumina sheets. However, unlike smectites, illite possesses interlayer potassium ions occupying vacant octahedral sites, resulting in stronger interlayer bonding and reduced swelling behaviour.
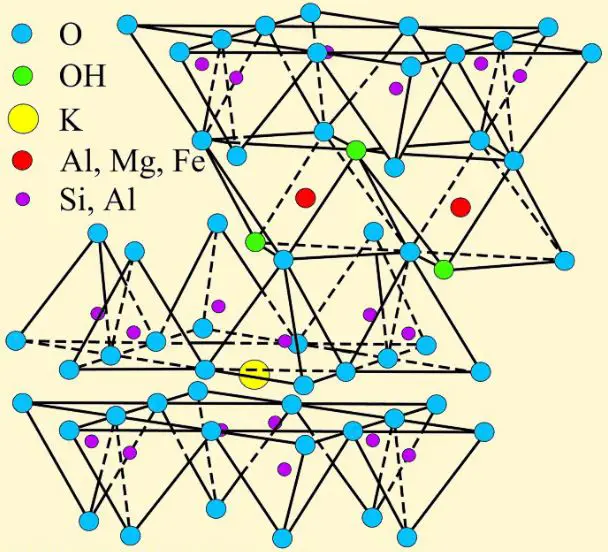
The illite particle has a similar structure to the montmorillonite particle. But in illite, the bond between the silica sheets at the bottom is made by potassium cations that are shared by nearby sheets. The potassium ions are the right size to fit into the gaps in the bottom of the silica sheet made by the tetrahedra. Sharing the potassium ions between the silica sheets makes a strong bond. So, the kaolinite and illite minerals are much less expansive than the montmorillonite.
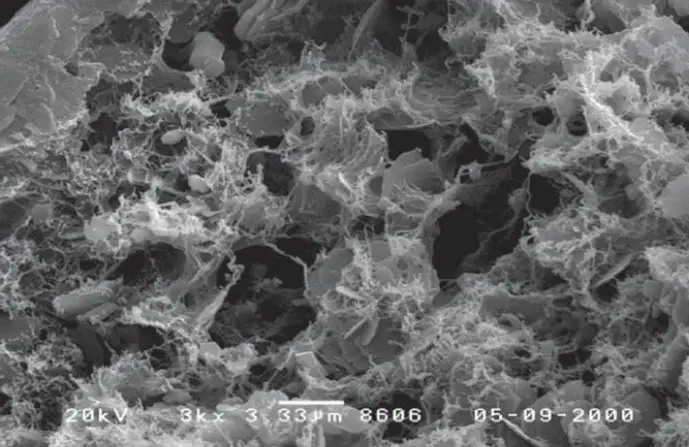
Characteristics of illite clay minerals
- Intermediate swelling behaviour: Compared to highly swelling smectites, illite exhibits minimal swelling upon hydration due to the presence of fixed interlayer potassium ions and stronger layer bonding.
- Moderate cation exchange capacity (CEC): While lower than smectites, illite possesses a notable CEC due to the exchange of cations on the basal plane and edges, influencing its interactions with various cations and organic compounds.
- High thermal stability: Due to its strong Si-O-Al bonds and stable interlayer potassium ions, illite exhibits exceptional thermal stability, making it suitable for high-temperature applications.
- Excellent rheological properties: The platy morphology and surface properties of illite contribute to its ability to thicken and suspend particles in aqueous suspensions, valuable for its use in drilling fluids and other industrial processes.