The nature of the soil, its plasticity, clay content, soil structure, and other factors can all be used to determine whether or not a soil has the potential to be expansive. Expansive soils must be identified during the reconnaissance and preliminary stages of a site investigation in order to determine the best sample and testing methods to use.
Expansive soils can cause considerable damage to civil engineering structures and foundations. This is due to the high swelling pressure they exert on foundations as they absorb water. Furthermore, their high shrinkage on drying can also affect foundations negatively.
The methods for determining the swell potential of expansive soils can generally be divided into two types. The first category mainly involves measurement of physical properties of soils, such as Atterberg limits, free swell, and potential volume change. The second category involves measurement of mineralogical and chemical properties of soils, such as clay content, cation exchange capacity, and specific surface area (Nelson et al, 2015).
To detect expansive soils, practicing geotechnical engineers often rely solely on physical property measurements. However, agricultural and geological practitioners routinely measure mineralogical and chemical parameters, and the engineering community should not overlook them.
Many of the methods of identification simply detect the presence of minerals with the capacity to expand. Physical factors such as in situ water content and density are not taken into account. As a result, they do not always determine whether or not the natural soil deposit is genuinely expansive, nor do they assess the potential for expansion. Nonetheless, they serve as important indicators of the need to dig more into the expansivity potential.
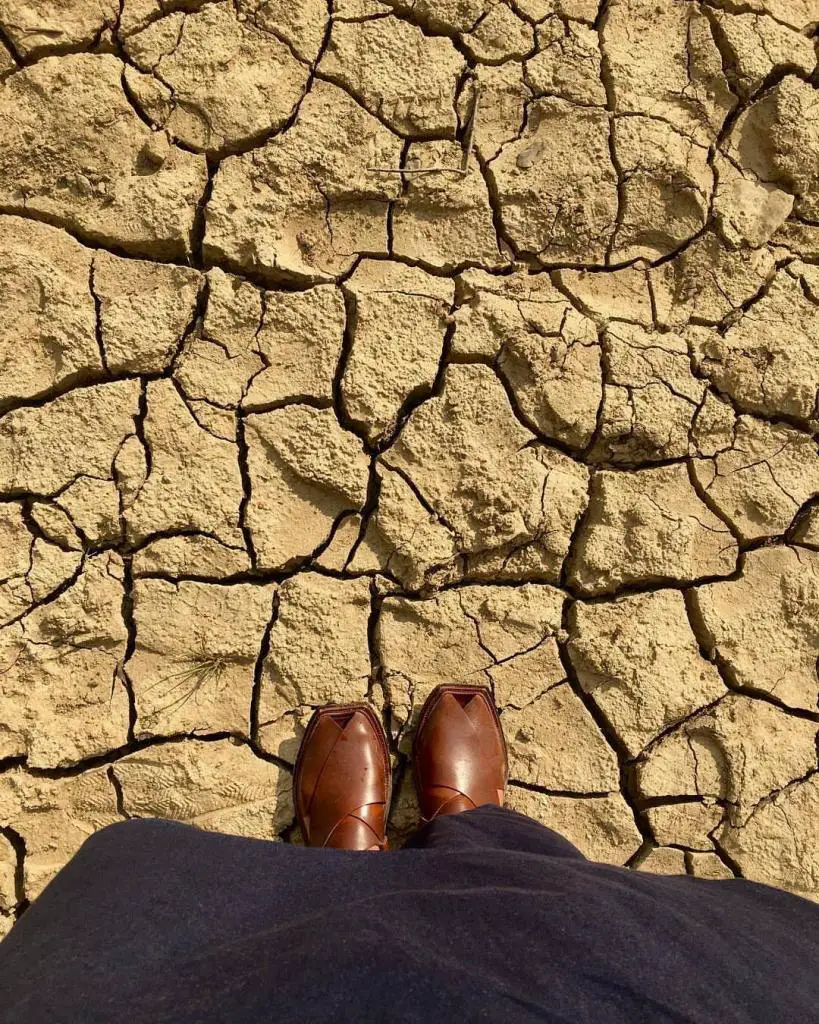
Identification of Expansive Soils Based on Physical Properties
These are the tests on the physical properties of soils that can be carried out in the laboratory to determine if the soil has the potential to be expansive. These tests are;
Methods Based on Plasticity
Expansive soils can be identified using the Atterberg limits. The plasticity index, PI, and the liquidity index, LI, are two indexes based on the Atterberg limits. One or both of these indices have been used in a variety of identification methods for expansive . More expansive minerals, on the whole, have a higher plasticity. According to Peck, Hanson, and Thornburn (1974), there is a general relationship between a soil’s plasticity index and its expansion potential, as illustrated in Table 1.
Table 1: Expansion Potential of Soils and Plasticity Index (Peck, Hanson, and Thornburn 1974)
Plasticity Index | Expansion Potential |
0 – 15 | Low |
0 – 35 | Medium |
22 – 55 | High |
> 55 | Very high |
However, Zapata et al. (2006) observed that the plasticity index alone does not accurately predict the expansion potential of remoulded expansive soils. They concluded that associating expansion potential with the product of plasticity index and % passing the No. 200 (75 μm) sieve improves the correlation significantly. It’s vital to remember that, while a soil’s plasticity may indicate the presence of expansive minerals, it’s not a guarantee that the soil is expansive.
Atterberg limits and clay content can be combined into a parameter called activity, Ac. This term was defined by Skempton (1953) as;
Activity = Plasticity Index / (% by weight finer than 2μm)
According to Skempton, clays can be divided into three categories based on their activity. “Inactive” for activities less than 0.75, “normal” for activities between 0.75 and 1.25, and “active” for activities greater than 1.25. The biggest potential for expansion is found in active clays. Table 2 shows typical activity values for a variety of clay minerals. The largest growth potential is seen in sodium montmorillonite, as evidenced by the extremely high value of activity in Table 2.
Table 2: Typical Activity Values for Clay Minerals (Skempton 1953)
Mineral | Activity |
Kaolinite | 0.33 – 0.46 |
Illite | 0.9 |
Montmorillonite (Ca) | 1.5 |
Montmorillonite (Na) | 7.2 |
Free Swell Test
The free swell test involves inserting a known volume of dry soil that has passed through the No. 40 (425 μm) sieve into a graduated cylinder filled with water and measuring the swollen volume after it has settled completely. The ratio of the change in volume from the dry to the wet condition over the initial volume, expressed as a percentage, is used to calculate the free swell of the soil.
The free swell value of a high-grade commercial bentonite (sodium montmorillonite) will range from 1200 to 2000 percent. According to Holtz and Gibbs (1956), soils with free swell values as low as 100 percent can expand significantly in the field when wetted under modest loading. In addition, according to Dawson (1953), certain Texas clays with free swell values in the 50 percent range have caused significant damage due to expansion. Extreme climate circumstances, along with the soil’s expansion tendencies, caused this.
The free swell index (FSI) is calculated as;
FSI = [(soil volume in water − soil volume in kerosene)/ soil volume in kerosene] × 100%
The expansion potential of the soil as classified according to the FSI is shown in Table 3.
Table 3: Expansion Potential Based on Free Swell Index
Free Swell Index (FSI) | Expansion Potential |
< 20 | Low |
20 – 35 | Medium |
35 – 50 | High |
> 50 | Very High |
Potential Volume Change (PVC)
T. W. Lambe (1960) developed the potential volume change (PVC) method for the Federal Housing Administration. Figure 2(a) shows the PVC apparatus, which has been used by many states in the United States of America. The test consists of placing a remoulded soil sample into an oedometer ring. The sample is then wetted and allowed to swell against a proving ring in the device. The pressure on the ring is given as the swell index, which is connected to qualitative ranges of possible volume change using the chart in Figure 2(a) (Lambe 1960).
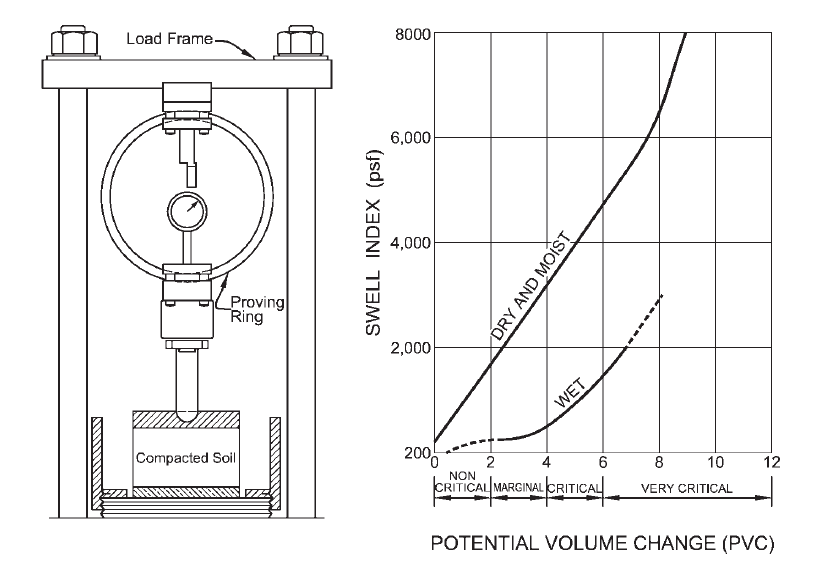
The test’s simplicity is a benefit. The downside is that the proving ring’s stiffness is not uniform, allowing for varying degrees of swelling depending on the stiffness of the proving ring. The quantity of swelling allowed by the proving ring will affect the swelling pressure that develops. The swell index and PVC values are more beneficial for identifying potential expansive behaviour and should not be utilised as design parameters for undisturbed in situ soils because the test uses remoulded samples.
Expansion Test (EI) Test
The expansion index test entails compacting a soil at a saturation level of 50% ± 2% under standard conditions. The sample is subjected to a vertical pressure of 144 psf (7 kPa) and then flooded with distilled water. Equation is used to calculate the expansion index, which is reported to the closest whole number.
EI = [(final thickness − initial thickness) / initial thickness] × 1000
The expansion potential of the soil is classified according to the expansion index, as shown in Table 4.
Table 4: Expansion Potential Based on Expansion Index
Expansion Index (EI) | Expansion Potential |
0 – 20 | Very low |
21 – 50 | Low |
51 – 90 | Medium |
91 – 130 | High |
> 130 | Very high |
The International Building Code (2012) and the International Residential Code (2012) both adopted the expansion index test for identification of an expansive soil. Both of the codes state the following:
Soils meeting all four of the following provisions shall be considered expansive, except that tests to show compliance with Items 1, 2, and 3 shall not be required if the test prescribed in Item 4 is conducted:
- Plasticity Index (PI) of 15 or greater, determined in accordance with ASTM D 4318.
- More than 10 percent of the soil particles pass a No. 200 sieve, determined in accordance with ASTM D 422.
- More than 10 percent of the soil particles are less than 5 micrometers in size, determined in accordance with ASTMD422.
- Expansion Index greater than 20, determined in accordance with ASTM D 4829.
Coefficient of Linear Extensibility (COLE)
The coefficient of linear extensibility (COLE) test measures the linear strain of an undisturbed, unconfined sample as it is dried from 5 psi (34 kPa) suction to oven-dry suction (150,000 psi = 1,000 MPa). A flexible plastic resin is applied to undisturbed soil samples during the procedure. The resin is impermeable to liquid water, but permeable to water vapor. Natural clods of soil are brought to a soil suction of 5 psi (34 kPa) in a pressure vessel.
Using Archimedes’ principle, they are weighed in air and in water to determine their weight and volume. After that, the samples are oven-dried, and another weight and volume measurement is done in the same way. COLE is a measurement of how much a sample’s dimension changes from wet to dry.
The value of COLE is given by:
COLE = ΔL∕ΔLD = (?dD∕?dM)0.33 − 1
where:
ΔL∕ΔLD = linear strain relative to dry dimensions,
?dD = dry density of oven-dry sample, and
?dM = dry density of sample at 5 psi (34 kPa) suction.
The value of COLE is sometimes expressed as a percentage. Whether it is a percentage or dimensionless is evident from its magnitude. COLE has been related to swell index from the PVC test and other indicative parameters. The linear extensibility, LE, can be used as an estimator of clay mineralogy.
The LE of a soil layer is the product of the thickness, in centimeters, multiplied by the COLE of the layer in question. The LE of a soil is defined as the sum of these products for all soil horizons. The ratio of LE to clay content is related to mineralogy as shown in Table 5.
Table 5: Ratio of Linear Extensibility (LE) to Percent Clay
LE/Percent Clay | Mineralogy |
< 0.05 | Kaolinite |
0.05–0.15 | Illite |
0.15 | Montmorillonite |
Standard Absorption Moisture Content (SAMC)
Yao et al. (2004) proposed the SAMC test as a means of identifying expansive soils. It was suggested in China’s Specifications for Highway Subgrade Design (CMC 2004). The SAMC test has the advantage of being simple. The SAMC is the water content at which a soil will reach equilibrium under specified conditions.
An undisturbed soil sample is placed on a porous plate within a constant humidity container above a saturated sodium bromide solution. After measuring the weight of the soil sample at equilibrium, it is oven-dried. The SAMC is determined as follows:
SAMC (%) = (We −Ws)/Ws
where:
We = weight of sample at equilibrium (77 ∘F and 60% relative humidity) and
Ws = weight of oven-dry sample.
China’s Specifications for Design of Highway Subgrades (CMC 2004) presents a method for classifying expansive soils based on the standard absorption moisture content, plasticity index, and free-swell values, as shown in Table 6.
Table 6: Classification Standard for Expansive Soils (CMC, 2004)
Standard Absorption Moisture Content (%) | Plasticity Index (%) | Free-Swell Value (%) | Swell Potential Class |
< 2.5 | < 15 | < 40 | Non-expansive |
2.5 – 4.8 | 15 – 28 | 40 – 60 | Low |
4.8 – 6.8 | 28 – 40 | 60 – 90 | Medium |
6.8 | > 40 | > 90 | High |
Mineralogical Methods
The presence of montmorillonite in a soil can be used to determine whether or not the soil is potentially expansive. A clay’s mineralogy can be determined based on its crystal structure or by chemical analysis. X-ray diffraction (XRD), differential thermal analysis (DTA), and electron microscopy are all popular mineralogical identification procedures.
The amount by which X-rays are diffracted around crystals is used to calculate basal plane spacing in XRD. DTA involves heating a sample of clay and an inert material at the same time. The resulting thermograms are contrasted to those for pure minerals, which are plots of temperature difference vs applied heat. On the thermograms, each mineral exhibits distinct endothermic and exothermic reactions. The clay particles can be observed directly using electron microscopy.
The size and shape of the particles can be used to make a qualitative identification. X-ray absorption spectroscopy, petrographic microscopy, soil micromorphology, digital image analysis, atomic force microscopy, and diffuse reflectance spectroscopy are some more mineralogical techniques (Ulery and Drees, 2008). In engineering practice, mineralogical approaches are rarely applied. They’re especially beneficial for research.
Chemical Methods
The most common chemical methods that are used to identify clay minerals include measurement of cation exchange capacity (CEC), specific surface area (SSA), and total potassium (TP). These methods are described in the following sections;
Cation Exchange Capacity (CEC)
The total amount of exchangeable cations required to balance the negative charge on the surface of clay particles is known as the CEC. Milliequivalents per 100 grammes of dry clay are used to calculate CEC. Excess salts in the soil are first eliminated, then adsorbed cations are restored by saturating the soil exchange sites with a known cation during the test procedure. For a mineral with a higher imbalanced surface charge, the amount of known cation required to saturate the exchange sites is greater.
Chemical examination of the extract can reveal the nature of the cation complex that was removed. Clay mineralogy is linked to CEC. A high CEC value suggests the presence of a highly active clay mineral like montmorillonite, whereas a low CEC value indicates the presence of a non-expansive clay mineral like kaolinite. In general, as the CEC rises, so does the expansion potential. Table 7 shows typical CEC values for the three most common clay minerals (Mitchell and Soga, 2005).
Table 7: Typical Values of CEC, SSA, and TP for Clay Minerals (Mitchell and Soga, 2005)
Clay Mineral | Cation Exchange Capacity (CEC) (meq/100 g) | Specific Surface Area (SSA) (m2/g) | Total Potassium (TP) (%) |
Kaolinite | 1 – 6 | 5 – 55 | 0 |
Illite | 15 – 50 | 80 – 120 | 6 |
Montmorillonite | 80 – 150 | 600 – 800 | 0 |
To determine the CEC of a soil, a variety of methods can be used. CEC measurement necessitates complex and precise testing procedures that are rarely performed in soil mechanics laboratories. However, many agricultural soils laboratories undertake this test on a regular basis, and it is rather inexpensive.
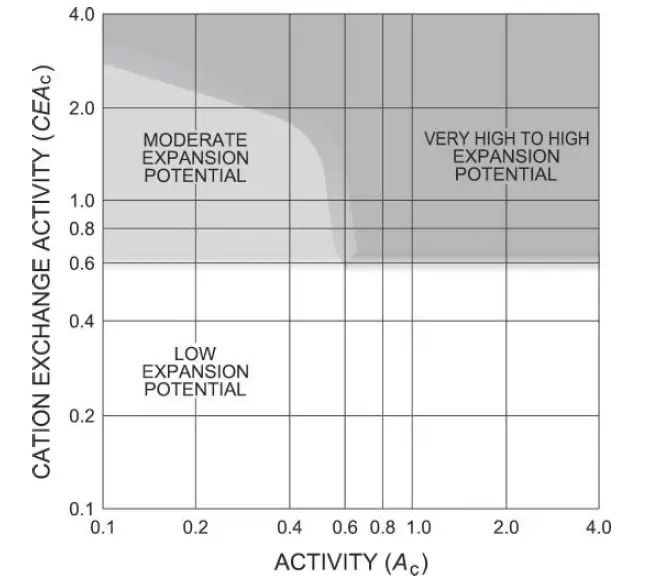
The graph in Figure 3 was created using data from the Natural Resources Conservation Service’s soil survey reports for soils in California, Arizona, Texas, Wyoming, Minnesota, Wisconsin, Kansas, and Utah. The CEAc (CEAc = CEC/clay content) versus Ac chart was used to create Figure 3, which was designed to be used as a generic classification method for potentially expanding soils.
Specific Surface Area (SSA)
The total surface area of soil particles in a unit mass of soil is described as the specific surface area (SSA) of a soil. The SSA of montmorillonite is substantially higher than that of kaolinite. Therefore, a clayey soil with a high SSA, will have a larger water holding capacity and better expansion potential (Chittoori and Puppala 2011).
A high SSA, on the other hand, does not always imply an expanding soil. If a soil has a high organic component, for example, that fraction may have a highly reactive surface with properties similar to those of a material with a large specific surface area (Jury, Gardner, and Gardner 1991). Several methods for determining a soil’s specific surface area have been devised. Adsorption of polar molecules, such as ethylene glycol, on the surfaces of clay minerals is the most prevalent approach.
The typical SSA values for the three basic clay minerals are shown in Table 7. The SSA of the montmorillonite minerals is around ten times that of the kaolinite group. Although within the same group of minerals, the range of typical SSA values indicated in Table 7 might vary by 100 percent or more, the variation in SSA between groups, notably for montmorillonite, is so great that mineral identification is usually attainable.
Total Potassium (TP)
The only clay mineral that includes potassium in its structure is illite. Therefore, the amount of potassium ions in a soil provides a direct indication of the presence of illite (Chittoori and Puppala 2011). Table 7 shows the differences in amount of total potassium between illite and the other two minerals. Thus, high potassium content is indicative of low expansion potential.
References
[1] China Ministry of Construction (CMC). 2004. “Specifications for the Design of Highway Subgrades.” JTG D 30, Beijing: Renmin Communication Press.
[2] Chittoori, B., and A. J. Puppala. 2011. “Quantitative Estimation of Clay Mineralogy in Fine-Grained Soils.” Journal of Geotechnical and Geoenvironmental Engineering, ASCE 137(11): 997–1008
[3] Dawson, R. F. 1953. “Movement of Small Houses Erected on an Expansive Clay Soil.” Proceedings of the 3rd International Conference on Soil Mechanics and Foundation Engineering, 1, 346–350.
[4] Holtz, W. G., and H. J. Gibbs. 1956. “Engineering Properties of Expansive Clays.” Transactions ASCE 121, 641–677.
[5] International Building Code (IBC). 2012. International Building Code. Falls Church, VA: International Code Council.
[6] International Residential Code (IRC). 2012. International Residential Code for One- and Two-Family Dwellings. Falls Church, VA: International Code Council.
[7] Jury, W. A.,W. R. Gardner, andW. H. Gardner. 1991. Soil Physics (5th ed.). New York: John Wiley and Sons.
[8] Lambe, T.W. 1960. “The Character and Identification of Expansive Soils, Soil PVC Meter.” Federal Housing Administration, Technical Studies Program, FHA 701.
[9] Mitchell, J. K., and K. Soga. 2005. Fundamentals of Soil Behavior (3rd ed.). Hoboken, NJ: John Wiley and Sons.
[10] Nelson J. D., Chao K. C., Overton D. D., Nelson E. J. 2015. Foundation Engineering for Expansive Soils. John Wiley & Sons, Inc
[11] Peck, R. B., W. E. Hanson, and T. H. Thornburn. 1974. Foundation Engineering (2nd ed.). New York: John Wiley and Sons.
[12] Skempton, A. W. 1953. “The Colloidal ‘Activity’ of Clays.” Proceedings of the 3rd International Conference on Soil Mechanics and Foundation Engineering, Switzerland, 1, 57–61.
[13] Ulery, A. L., and L. R. Drees. 2008. “Mineralogical Methods,” In Methods of Soil Analysis, Part 5. Madison, WI: Soil Science Society of America.
[14] Yao, H. L., Y. Yang, P. Cheng, and W. P. Wu. 2004. “Standard Moisture Absorption Water Content of Soil and Its Testing Standard.” Rock and Soil Mechanics 25(6): 856–859.
[15] Zapata, C. E., S. L. Houston, W. N. Houston, and H. Dye. 2006. “Expansion Index and Its Relationship with Other Index Properties.” Proceedings of the 4th International Conference on Unsaturated Soils, Carefree, AZ, 2133–2137.
[16] Zheng, J. L., R. Zhang, and H. P. Yang. 2008. “Validation of a Swelling Potential Index for Expansive Soils.” In Unsaturated Soils: Advances in Geo-Engineering, edited by D. G. Toll, C. E. Augarde, D. Gallipoli, and S. J. Wheeler. London: Taylor and Francis Group.