Pykrete is a material used for temporary buildings in cold climates. It is made of a mixture of water and a number of additives, such as wood chips, cellulose sheets that have been dissolved, sand, gums, and combinations of any of those. It was initially created so that ships could be built in cold environments. In the past ten years, this substance has typically been used in shell buildings that have been built with ropes and inflatable fabric formwork (see Figure 1).
The usage of Pykrete in linear element constructions has recently been studied, and some low-rise structures have been constructed as a result. According to Pronk et al (2022), the year 2019 saw the construction of a tower-like Pykrete structure for the International Ice and Snow Innovation Design and Construction Competition, based on an idea from the Eindhoven University of Technology. It is the tallest pykrete structure with linear elements to date, this structure has a height of 11 m.
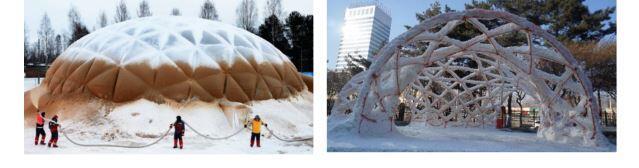
Researchers (Pronk et al, 2022) from the Department of the Built Environment, Eindhoven University of Technology, Netherlands have carried out a study on the mechanical performance of pykrete beam elements. Experimental tests from the study were compared with previously conducted studies. Furthermore, the researchers presented the optimization and numerical model of a pykrete tower’s design, followed by a description of the construction techniques. The article was published in the journal, Structures (Elsevier).
Making and Design Concept of the Lattice Tower
In the study conducted by Pronk et al (2022), a preliminary design of the tower was developed, considering a uniform cross-section for the primary elements and secondary elements. The preliminary design of the tower was symmetrical, made up of five similar partitions.
Ten principal load-bearing parts, five of which are exterior (shown in blue in Figure 2) and five of which are internal, extend vertically from the bottom to the top (green elements in Figure 2). Self-weight and wind pressure of 0.5 kN/m2 were taken into account. Recent research literature served as the source for the material’s mechanical properties.
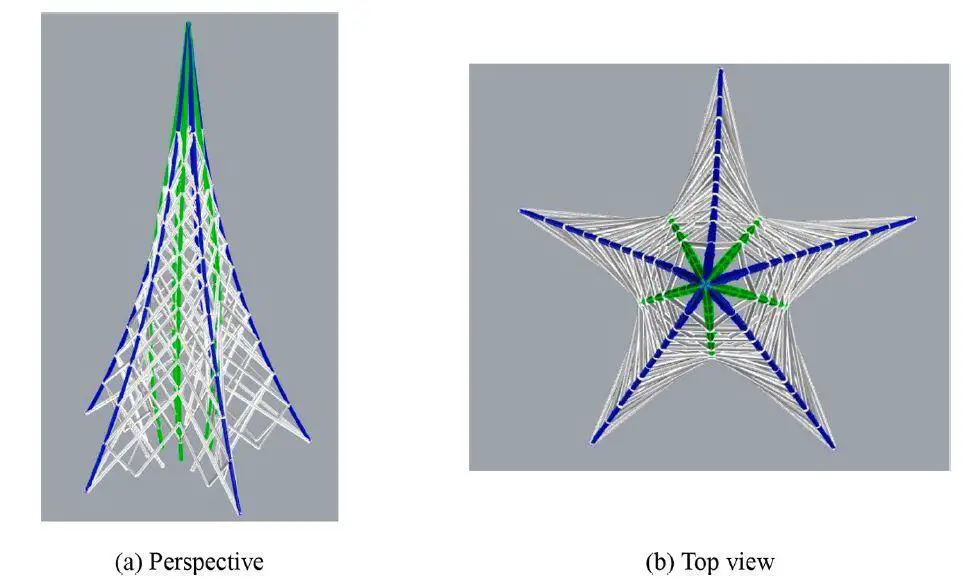
Fire hoses filled with pykrete were used to create the members of the lattice tower. They transfer the wind loads in addition to the dead weight of the structure to the foundations. Compression in the inner columns is primarily produced by the structure’s self-weight. Due to the additional weight of the structural elements, these compressive forces are low at the top of the tower and increase at ground level, as typical in all structures. As a result, it is anticipated to have a larger cross section close to the foundation.
According to the research, the tower’s construction was separated into three stages:
- Preparing the rope and pipes: The ropes and fire hoses are trimmed to the correct length. Pykrete is injected into the fire hoses from one side. The hoses were linked together after being frozen. The secondary components, the ropes, are tied together and attached to the fire hoses in accordance with the prescribed pattern. The anchors are made of earth connectors. The hoses are hermetically sealed below ground. To keep the shape while the pykrete is being applied, a low tension is applied to these ropes.
- Pipe and rope installation without the use of Pykrete: Here, the structure is raised and positioned using a crane (see Figure 3).
- Application of pykrete: As soon as the necessary sections are achieved, the pykrete will be gradually applied by spraying and extrusion on the hoses and ropes. Pykrete can be sprayed on the structure until the desired member thickness is achieved after the desired form has been achieved. The top portion, which is currently being lifted by the crane, will be sawn off once enough pykrete has been put, and the tower will then stand on its own.
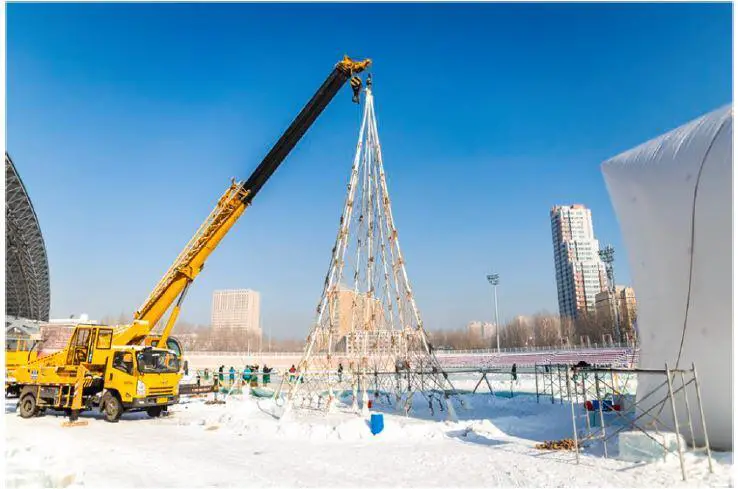
Based on the preliminary tower design, two main types of samples were evaluated. The cross-section of the first kind of sample is made up of pykrete and a fire hose. A tube with a 64 mm diameter was used as the hose. Pykrete is poured into the tube, and a 44 mm thick second layer of pykrete is applied around the tube, making the section’s overall diameter 154 mm. The mixture of additives in Pykrete contains cellulose at a concentration of 20 g/L (2%).
The second type of sample, which has a smaller cross-section was used to test for secondary elements. It was made up of a rope with a diameter of 12 mm that is encircled by a layer of pykrete measuring 15 mm. The additive concentration is 80 g/L (8%).
The greatest length that can be tested corresponds to a sample length of 450 mm for both types of samples. Testing was done using three different methods: compression, tension, and 4-point bending. For pykrete samples, there aren’t any currently available standardised tests. It is important to understand that pykrete’s mechanical characteristics vary depending on temperature.
Mechanical Performances of Pykrete in Beam Elements
In comparison to common building materials, pykrete’s mechanical characteristics are still being extensively studied. Tests have been done on a large number of samples in order to compare different pykrete compositions. The force–displacement relationship obtained by the bending and compression tests in the current study generally followed the major trends already observed in previous studies. However, some of the takeaways from the study were;
- Regarding the 4-point bending tests, it appears that the fire hose behaviour during bending shows a hardening phase. The authors however recommended that further research should examine additional samples with composite sections to corroborate this.
- The compression tests revealed that when using slender linear elements, which weren’t taken into account for shell structures in Pykrete, it will be required to pay attention to buckling.
- The tests conducted on the fire hose sections produced an elastic modulus that is relatively low and especially much below what is described in the literature. Although the cause of this outcome is unclear, a lack of uniformity in the parts may be to blame.
- The tensile tests reveal that while the rope does not increase the section’s tensile strength, it does allow for the preservation of the element’s integrity after failure, which is not possible for an element constructed simply of pykrete.
The full results of the test data are available in the publication.
Optimization and Numerical Model of the Tower
Grasshopper® software was used to optimize the tower’s design based on the results of the investigation. It is a Rhino® visual programming plugin that enables the execution of parametric designs based on scripts. A Live Physics engine called Kangaroo2® allows for interactive simulation, optimization, and form-finding within Grasshopper. Kangaroo2® uses dynamics relaxation With this approach, a nonlinear equation system’s solution is reduced to an explicit iterative calculation. Therefore, a damped dynamic process led to the proposed static solution.
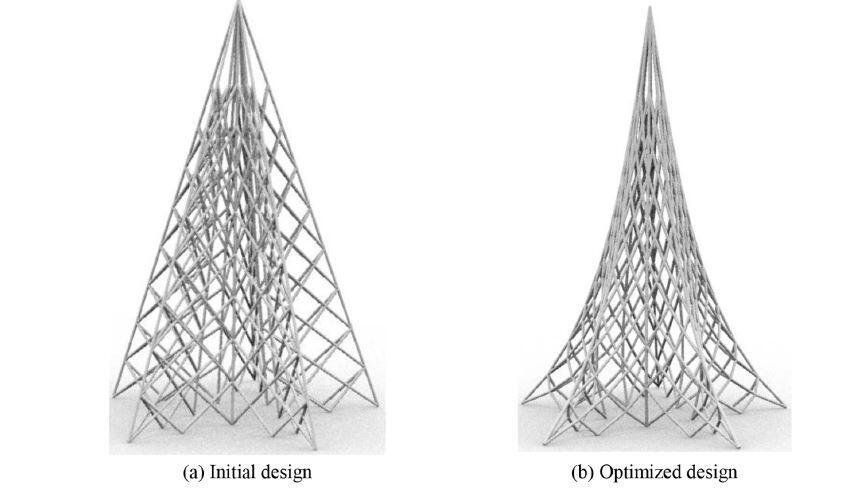
The algorithm consists of the subsequent phases. The anchor points, the self-weight, the major element stiffness, and the secondary element stiffness are first defined as the constraint conditions. Then, utilizing Newton’s second law, masses are defined at each node. The total residual forces for each node, the speed, and the position are computed at each iteration. When the geometry reaches its static state, the algorithm will terminate.
The optimum design consists of a series of curves that can be connected to form a mesh. In other words, the gradient of the energy that is in the direction of the forces (masses) defined at each node is used to move the nodes in order to minimize the elastic energy.
The next step is to assess the design’s structural performance in SCIA® under wind loads and self-weight. The elements’ cross-sections are manually modified (decreased or increased). For the primary and secondary parts, there can only be three different sections. Finally, the original design and the new cross-sections are added as a new input in Grasshopper®, and Kangaroo2® generates a new shape.
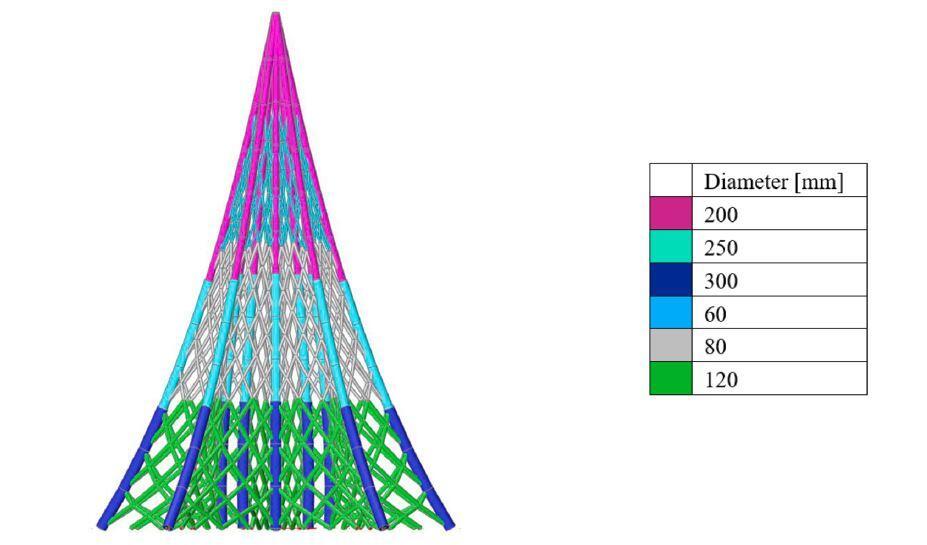
Conclusion
Pykrete is a promising and environmentally friendly material for constructing buildings in cold areas. Up until now, inflatable shell structures were the only types of pykrete structures. However, this restricts the kind of constructions that can be made to the shapes of inflatables that can be built.
According to the study, producing truss structures would present new opportunities. By recommending a construction method and performing testing on the sections, the research enabled the creation of an 11 m tall tower made up of linear elements. By using form-finding, the design was optimized, and SCIA® was used to verify the structure under actual loading. The experimental testing have demonstrated that, before constructing more ambitious structures, research on the buckling of these components is required.
Reference(s)
Pronk A., Mergny E., and Li Q. (2022): Structural design of a lattice pykrete tower. Structures 40 (2022) 725-747. https://doi.org/10.1016/j.istruc.2022.03.079
The contents of the cited original article published by Structures (Elsevier) is open access, under the CC BY license (http://creativecommons.org/licenses/by/4.0/) which allows you to share and adapt (remix) the article provided the appropriate credit is given, and the link to this license provided.