Vibration issues experienced in buildings are frequently attributable to deficiencies in the structural system. Consequently, vibration analysis has become an integral component of structural design, especially in structural elements that are susceptible to vibration. This can be achieved by carrying out a dynamic analysis of the structure.
For instance, the increasing prevalence of long-span and lightweight floor systems has led to more problems of floor vibration in buildings. These kinds of structures are characterized by lower natural frequencies and reduced damping and have necessitated increased attention to the dynamic behaviour of floors under human activities.
Floor vibrations may originate from external sources such as vehicular traffic. To mitigate such disturbances, isolating the entire building is often recommended. However, pedestrian traffic represents the most common and significant internal source of dynamic excitation.
Rhythmic pedestrian movement imparts periodic forces to the floor, potentially inducing amplified responses. Consequently, structures intended for pedestrian activity must not only possess adequate strength but also adhere to comfort and vibration serviceability standards.
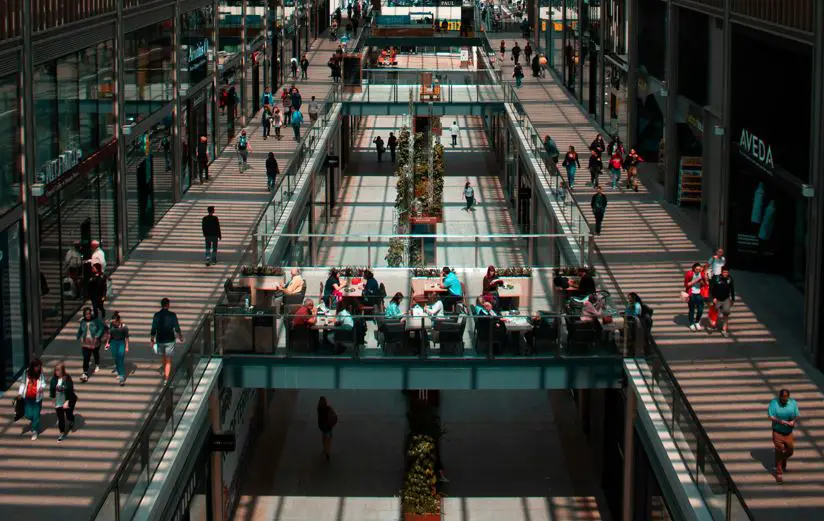
Human sensitivity to building or floor vibration varies. While individuals can detect even subtle vibrations, significant increases in vibration amplitude often result in comparatively minor changes in perceived intensity. Although floor vibration can engender feelings of discomfort or insecurity, it is important to emphasize that the vibration of a floor in a building does not inherently mean that structural safety is compromised.
Retrofit measures to attenuate floor vibration in existing structures are typically impractical due to the necessity for substantial modifications to the floor system’s mass, stiffness, or damping properties. Consequently, establishing acceptable vibration levels during the initial design phase, with careful consideration of anticipated floor use, is imperative.
Therefore, proactive identification and mitigation of potential vibration problems are most effectively achieved during the preliminary design phases. By incorporating vibration considerations at this juncture, engineers can make informed decisions to optimize structural performance and preclude costly remedial measures.
This article considers the design considerations to be made during the preliminary analysis of the vibration of floors.
Damping
Damping is a process by which energy is dissipated and/or dispersed (hysteresis) from a body, thereby attenuating vibrations over time. Structural damping originates from factors such as joint friction, slip, and the presence of furnishings and fixtures, which absorb vibrational energy through their own movement. Given the variability of these factors across and within buildings, design decisions should rely on historically validated damping values.
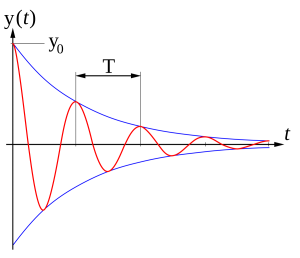
In practical applications, the following critical damping ratios (ζ) are commonly adopted for design purposes in typical steel-framed structures. These values should be employed unless more precise data is accessible.
Damping ratio ζ (%) | Floor finishes |
0.5% | For fully welded steel structures, e.g. staircases |
1.1% | For completely bare floors or floors where only a small amount of furnishings are present. |
3.0% | For fully fitted out and furnished floors in normal use. |
4.5% | For a floor where the designer is confident that partitions will be appropriately located to interrupt the relevant mode(s) of vibration (i.e. the partition lines are perpendicular to the main vibrating elements of the critical mode shape). |
While damping values for unoccupied, bare floors are infrequently utilized in design due to the impracticality of such conditions, assessing performance under these circumstances offers value to engineers. Anticipating potential criticisms regarding floor acceptability prior to full occupancy necessitates a preliminary evaluation of the bare floor’s vibrational characteristics.
Floor Loading
Accurate representation of mass distribution on the floor of the building is important for reliable vibration analysis. Increased floor mass reduces floor response at specific frequencies. Therefore, design calculations should employ unfactored self-weight, incorporating superimposed dead loads such as ceilings and utilities, unless a bare-structure analysis is required.
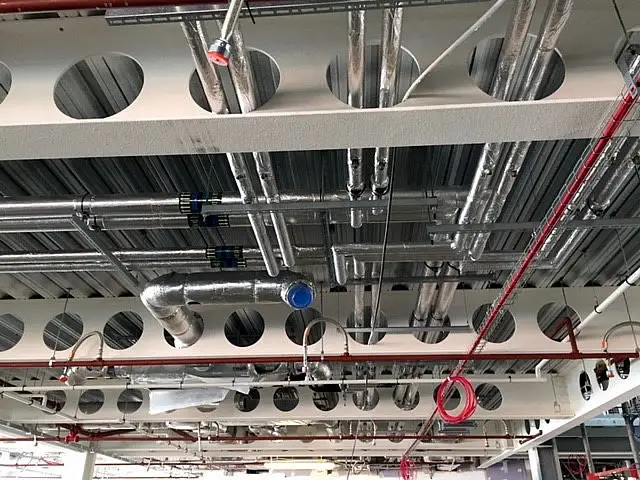
The assumption of uniformly distributed loading may not accurately represent actual floor conditions. Consequently, careful consideration should be given to load distribution patterns within the intended floor space. While an overall average load can be reasonably estimated, specific areas, such as storage spaces, may experience significantly higher loads.
In such cases, employing the heavier load for natural frequency calculations and the lighter load for response determination provides a conservative design approach. However, utilizing precise load distribution data through methods like finite element analysis can yield less conservative and more accurate results.
In cases where semi-permanent loads are assured within the completed structure, their inclusion is permissible; however, this practice should be excluded for dance or aerobic floors.
The UK National Annex to EN 1990 stipulates a 30% imposed load factor for serviceability limit state calculations in offices and residential buildings. However, this provision may be overly conservative for vibration analysis due to contemporary trends towards open-plan layouts and reduced physical documentation.
The discrepancy between design imposed loads and actual occupancy conditions suggests that considering only permanent imposed loads, or even neglecting imposed loads entirely as a conservative approach, may be more appropriate. Research by Hicks et al. recommends limiting the imposed load allowance to 10% of the nominal value.
Dynamic Modulus of Elasticity of Concrete
The calculation of natural frequency should utilize the dynamic modulus of elasticity for concrete. Recommended values are 38 kN/mm² for normal-weight concrete (approximate dry density: 2350 kg/m³) and 22 kN/mm² for lightweight concrete (approximate dry density: 1800 kg/m³).
Structural and Floor Configurations
Steel-concrete composite floors
Steel-concrete composite floor construction involves the integration of steel beams and concrete floor slabs through the use of shear connectors. These connectors facilitate composite action by transferring longitudinal forces between the steel and concrete components. The secondary (floor) beams are typically supported by primary (main) beams, which constitute the primary structural framework of the building.
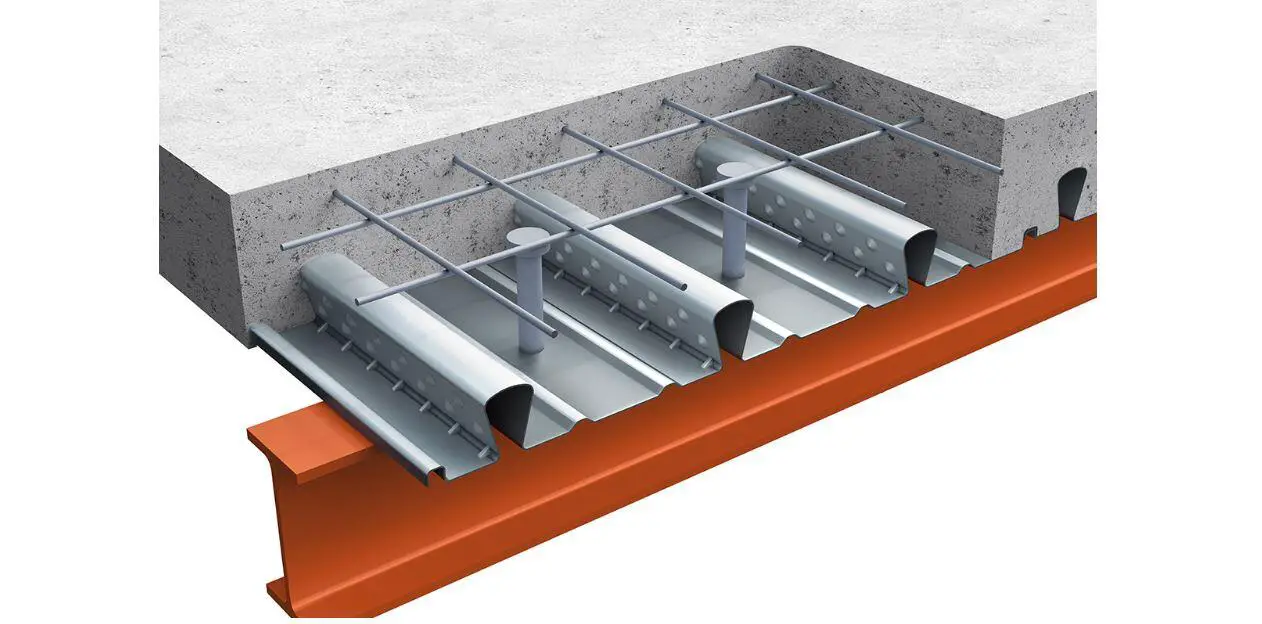
It is important to note that the deflection and stress levels associated with tolerable dynamic responses are minimal, with dynamic stress amplitudes typically less than 1% of static design stresses. Given these low-stress levels, the conventional assumption of simply supported beams and slabs may not be entirely accurate. In many cases, insufficient strain exists to overcome frictional forces, leading to structural behaviour resembling continuous beams, even when not explicitly designed as such.
Cantilevers
While cantilever construction is relatively infrequent, the natural frequencies can be obtained by employing the equation below.
fn = (kn/2π) × √(EI/mL4)
where:
EI is the dynamic flexural rigidity of the member (Nm2)
m is the effective mass (kg/m)
L is the span of the member (m)
Kn is a constant representing the beam support conditions for the nth mode of vibration. For a cantilever, n for the first mode may be taken as 3.52.
Nevertheless, due to the suboptimal mobilization of mass when dynamic excitation is applied near the cantilever’s free end, employing the simplified guidelines for response evaluation may yield conservative results. It is recommended to utilize the full finite element modelling and analysis for the response evaluation of cantilever floors.
Light Steel Frame Floors
The prevalence of light steel framing and modular construction has significantly increased over the past decade. This system is particularly popular in residential building applications, and much of the design guidance provided herein is tailored to this context.
Light steel floors are defined as those constructed with support members possessing a second moment of area not exceeding 450 cm⁴ and floor coverings such as timber boards, chipboard, plywood, or cement particle board. Given the anticipated high natural frequency of these systems (f1 ≥ 8 Hz for dwellings and f1 ≥ 10 Hz for corridors), impulsive responses to pedestrian traffic will predominate.
Thin Floors With No Internal Columns
In certain instances, floor dimensions or structural simplicity may necessitate a plate-like analytical approach rather than a discrete floor element model. These conditions typically arise in structures devoid of internal columns, where floor beams extend uninterruptedly between exterior columns.
Floor Response and Structural Behaviour
The magnitude of a floor’s vibrational response is influenced by the mass participating in the dynamic movement. To optimize performance, designers can manipulate the extent of this participating area through two primary strategies:
- Maximizing Participative Area: By enhancing floor plate continuity, a larger mass is engaged in the vibrational response, thereby reducing its amplitude.
- Isolating Critical Areas: Separating sensitive areas from regions prone to significant vibration can mitigate discomfort or disturbances.
A floor designed as statically discontinuous may exhibit continuous behaviour under dynamic conditions. If a floor slab spans multiple beams or benefits from structural continuity provided by other elements, it can generally be considered continuous for dynamic analysis. However, structural design considerations also impact dynamic performance.
For instance, in composite beam applications, inadequate transverse reinforcement can lead to progressive cracking and reduced continuity, thereby deteriorating vibrational performance over time. Conversely, floors lacking continuity should be analyzed as independent simply supported slabs, resulting in smaller participating areas and potentially amplified responses.
While floor continuity enhances structural performance, it can inadvertently amplify vibrations in unintended areas, potentially exceeding acceptable thresholds. In instances where vibrational disturbances from specific activities are likely to impact sensitive spaces, isolating the source of the activity is recommended.
This can be achieved by structurally separating the affected area from the remainder of the floor through the implementation of construction joints along its perimeter. Such isolation effectively prevents vibrational transmission and is particularly beneficial in environments with stringent vibration limitations, such as operating theatres.
An alternative approach to isolating a floor involves increasing its local stiffness. This method offers the advantage of controlling the sensitive area without necessitating modifications to the overall floor design. By enhancing stiffness, the region becomes effectively isolated from the remainder of the floor. Typically, achieving this requires a thicker floor slab, which should be considered during design to accommodate necessary headroom and service installations.