Is maintaining optimal safety levels in your workplace or facility your main priority? Installing guard rail kits correctly plays an essential role in accident prevention while maintaining safety regulation compliance. According to recent statistics, workplace accidents show a rising trend, while many incidents could be avoided through the implementation of effective safety barriers.
The correct installation of appropriate guard rail kits can significantly lower accident rates while establishing secure workspaces for all.
What You’ll Learn Today:
- Understanding Guard Rail Kit Basics
- Pre-Installation Planning Essentials
- Essential Tools and Materials: Your Installation Toolkit
- Step-by-Step Installation Guide
- Common Installation Mistakes to Avoid
- Maintenance and Safety Compliance
What Are Guard Rail Kits Really About?
Guard rail kits serve as life-saving systems engineered to protect both people and property against potential dangers beyond being mere metal barriers.
Guard rails serve as critical safety components in warehouses and loading docks alongside parking structures where vehicles may share space with pedestrians and valuable equipment. The right guardrail system can prevent:
- Vehicle collisions with structures
- Falls into dangerous areas
- Equipment damage
- Pedestrian injuries
- Costly property destruction
Proper knowledge of quality guard rail system requirements is critical before beginning installation steps. The best guardrail systems combine durability, proper materials, and careful installation to create a reliable safety barrier. Choose components that either fulfil or surpass OSHA guidelines and industry standards.
The success of your guard rail entirely depends on how well it is installed. A system with the highest quality will fail to perform properly if it is not installed correctly.
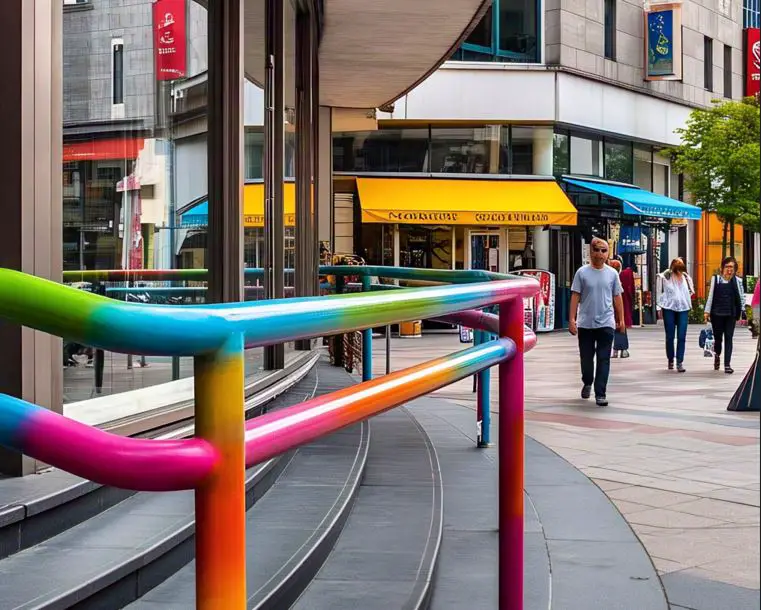
Types of Guard Rail Kits: Choosing What’s Right
Different guard rail kits possess varied levels of quality. The initial step in achieving a successful installation begins with choosing the type that best meets your requirements.
The U.S. railing market reached a valuation of $5.92 billion in 2022 and is expected to reach $8.04 billion by the year 2030. Because of their durability and minimal maintenance requirements metal railings will expand at a CAGR of 4.2%.
Steel guard rails protect high-impact areas while modular systems adapt easily to changes, and industrial rails serve warehouse environments, along with removable guard rails, which provide temporary access points.
Pre-Installation Planning: Setting Yourself Up For Success
Are you familiar with the adage “measure twice before cutting once”? That applies doubly to guard rail installation. When safety depends on it proper planning avoids poor performance.
Complete these critical planning steps before you start using any tools.
- Site Assessment: Evaluate the area for obstacles
- Measurement: Accurately measure the installation area
- Layout Planning: Mark locations for posts and rails
- Utility Check: Ensure you won’t hit utilities when drilling
- Compliance Check: Verify your plan meets safety codes
Here’s something many installers miss…
The distance between posts must be precise because excessive spacing weakens the whole system. Always follow manufacturer guidelines for maximum spacing.
Essential Tools and Materials: Your Installation Toolkit
Preparing your tools beforehand will expedite your installation process while reducing frustration and guaranteeing correct installation.
Standard guard rail kit installation requires the following tools and materials:
- You need a hammer drill with masonry bits, together with an impact driver for installation.
- You’ll need a measuring tape along with levels, a chalk line and a wrench set for installation.
- Safety equipment (glasses, gloves, steel-toe boots)
- Concrete anchors appropriate for your surface
- Shims for levelling and touch-up paint
But here’s the thing most people forget…
Maintain a supply of additional anchors, bolts, and nuts at all times. Installation progress will be impeded when you run out of fasteners during the process.
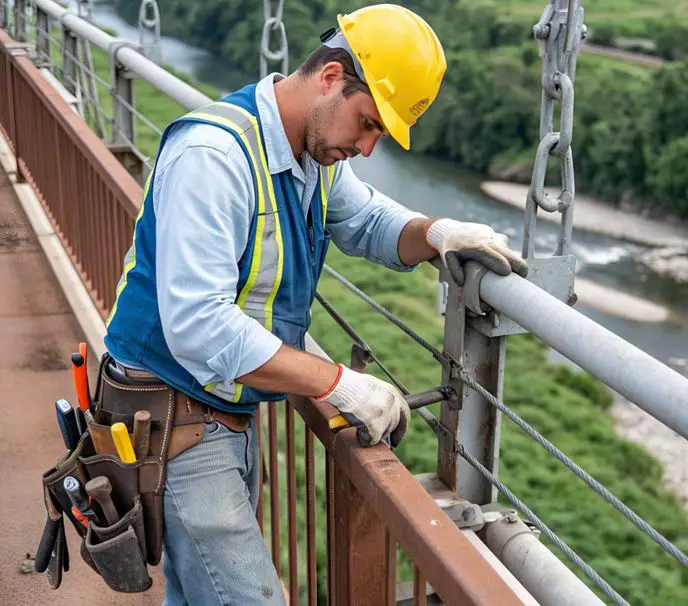
Step-by-Step Installation Guide: Doing It Right The First Time
The primary task begins here with the correct installation of your guard rail kit. Ensure your installation is safe and secure by following these steps precisely.
1. Prepare The Area
- Clear the installation zone of obstacles
- Sweep or clean the mounting surface
- Mark your layout with chalk lines
- Double-check measurements
2. Position The Posts
- Mark anchor locations for each post
- Drill pilot holes according to anchor specifications
- Install posts in their designated positions and verify that they stand perfectly vertical.
- Secure posts loosely – don’t fully tighten yet
Pro tip: A cardboard template helps ensure anchor holes are placed consistently on each post.
3. Attach Rails To Posts
- Align the first rail section with posts
- Insert connection hardware loosely
- Check the level and height
- Proceed to connect remaining rail sections
- Ensure proper overlap at connections
4. Final Alignment and Tightening
- Double-check the entire system for alignment
- Check that rails maintain a uniform level while keeping consistent height throughout.
- Ensure posts are perfectly plumb
- Systematically tighten all connections to specified torque
- Recheck alignment after tightening – adjust if needed
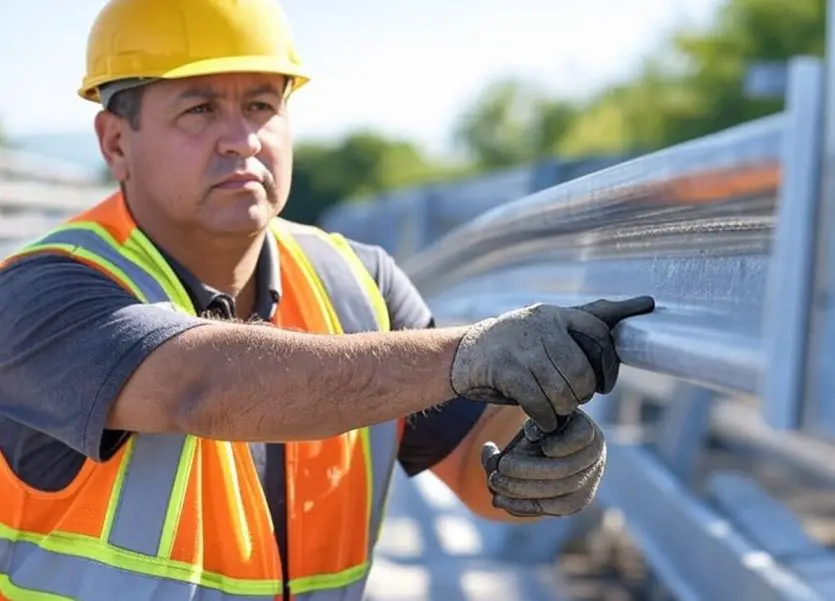
This is critical: Components may be damaged by over-tightening while system failure results from insufficient tightening. Use a torque wrench when possible.
5. Finishing Touches
- Use touch-up paint to fix any scratches or cut edges.
- Remove all debris from the installation area
- Perform a final inspection of all components
Did you know?
The Federal Railroad Administration allocated more than $66 billion during 2022 to 2026 for rail infrastructure projects which include improvements to crossings and safety barriers. This topic focuses on railway safety yet shows how important it is to install barrier systems correctly.
Common Installation Mistakes: Avoid These Pitfalls
The reasons behind guard rail installation failures can be understood by examining common errors. These frequent installation errors are simple to commit yet may bring about severe negative outcomes.
- The failure of guard rail systems often results from installing anchors that do not match the surface requirements.
- The entire system becomes weaker when posts are spaced too far apart.
- Poor Installation Practices: Fails to verify that posts stand perfectly vertical and that rails maintain a consistent level.
- Inadequate Torque: Not tightening fasteners to specifications
- Overlooking inspections during the installation process and after completion.
Take your time with each step. Guard rail systems that are installed correctly will remain effective for many years, but systems that are rushed through installation may malfunction precisely when you need them most.
Maintenance and Compliance: Keeping Your Guard Rails Safe
The installation of your guard rail kit requires ongoing maintenance and compliance with standards to maintain its effectiveness.
Your maintenance routine should include scheduled inspections to visually check for damage while verifying hardware tightness and confirming alignment. Guard rail systems function as insurance policies because, while you hope they remain unnecessary, they must perform flawlessly when activated.
The installation of your guard rail system needs to meet the height, strength, and placement standards set by OSHA Requirements, along with the regulations of local building codes. Ignoring guard rail compliance exposes facilities to inspection failures and financial penalties while raising liability risks and insurance problems.
Improvements to the Railway-Highway Crossing Program resulted in a 41% decrease in railway-highway crossing fatalities throughout the period from 2000 to 2023. Proper installation of safety barriers and adherence to safety standards demonstrate their life-saving potential.
The Bottom Line: Safety That Lasts
When guard rail kits are installed correctly, they serve as a long-term safety investment that safeguards both people and property for many years.
Remember these key points:
- Identify and select the suitable guard rail system based on your specific requirements
- Plan thoroughly before starting installation
- Follow the installation procedures step by step
- Avoid common installation mistakes
- Maintain and inspect regularly
The current emphasis on safety infrastructure demonstrates a response to urgent requirements rather than being a fleeting trend. The necessity for proper installation of guard rail systems in highways, roads, bridges, parking lots, and industrial locations has reached its peak importance.
This guide helps you construct a safety system designed to deliver optimal protection during critical situations.